Since her student days, Radhika Nagpal has been pondering one of biologyÍs most compelling questions: How can a small group of cells grow and assemble into a healthy baby? But since sheÍs not a biologist, she asks the question a different way: ñHow can an embryoÍs growth teach us to make more powerful computing systems?î
ñWith an embryo, thereÍs so much that can go wrong,î said Nagpal, an assistant professor of computer science in HarvardÍs School of Engineering and Applied Sciences. ñIndividual cells will die or be killed and yet the embryo recovers. And without any clear leader, embryonic cells solve a whole cascade of problems, such as where to put the head or the stomach and how to make their sizes match.î
Nagpal specializes in a field called amorphous computing, which uses inspiration from collective behavior in nature – such as how social insects cooperate with each other in a colony – to design collective behavior in computer networks and robot swarms.
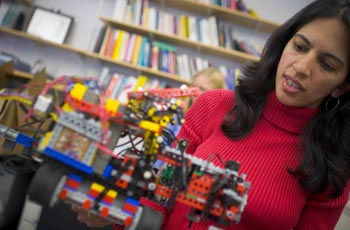
Originally from the city of Amritsar in India, Nagpal came to Harvard in 2003, after completing her Ph.D. at MIT. She is both a computer scientist who studies collective interactions in biology, and a math whiz who reduces those interactions to computational algorithms. At the Wyss Institute, her studies have led to major advances in robotics and computer networks, with the potential for applications in medicine and architecture.
Animated and quick to laugh, Nagpal describes nature as a source of creative ideas. ñI can look at a colony of ants and ask ïcan these insects show me how to build a better robot?î
Nagpal has shown through her work that the answer is ñyes.î To her, individual ants, or cells in a developing embryo, are parts of a larger, cooperative venture. A single cell does just a few things, but collectively, trillions of them can make a human being. Likewise, a lone ant is insignificant compared to the colony it lives in. ñNo one individual can see very far and none of them can talk to everyone at once,î Nagpal explained. ñSo nature uses strategies to extract as much as possible from these limited components.î
These groups are robust and self-healing, a resilience that can be expressed mathematically, with rules that translate a groupÍs objectives into instructions for its members. NagpalÍs goal is to figure out those rules and then use them to engineer modules each limited in terms of its own computational power that cooperate around a particular task.
NagpalÍs robots are made from such independent, connected, and programmable modules, which work together to adapt to environmental changes. Just as migrating birds can change course to avoid a storm, NagpalÍs modules can sense local changes and compensate with actions that adjacent modules respond to. So, a self-balancing table built by her graduate student Chih-Han Yu can perch on four robotic legs that bend and move as the ground sways. This table can rest on a tilting surface and balance a glass of water without spilling a drop. Such technology could someday be used to build a robotic bridge that stays level during earthquakes, Nagpal said.
Inspired by social insects, Nagpal and her collaborator, Wyss Faculty member Robert Wood, are also designing robotic ñswarmsî that perform group tasks, such as crawling into pipes to repair cracks. They will be capable of changing their shape – to negotiate curves in the pipe for instance _ inspired by how cells move and change shape in the body. Such robots could also be programmed to hone in on diseased arteries, and aggregate into stents that improve blood flow without surgery.
Nagpal says she embraces natureÍs imperfections, as much as its potential. ñCells donÍt move according to a synchronized plan,î she says. ñThereÍs some randomness to what they do, they arenÍt always reliable. The same applies in engineering – I canÍt build a perfect robot thatÍs going to work all the time. What I want is for the robot to be predictable in spite of its faults, and thatÍs what excites me about biology. Organisms can make it even when things go wrong, because they make robust decisions. So IÍm always looking for the hidden ideas in biology. Once we find them, we can engineer things we didnÍt think were possible before.î