Self-powered, homeostatic nanomaterials that actively self-regulate in response to environmental change
Living organisms have developed sophisticated ways to maintain stability in a changing environment, withstanding fluctuations in temperature, pH, pressure, and the presence or absence of crucial molecules. The integration of similar features in artificial materials, however, has remained a challenge—until now.
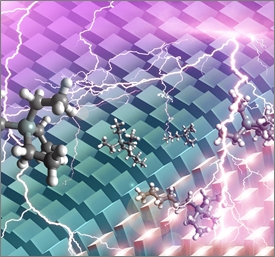
In the July 12 issue of Nature, a Harvard-led team of engineers presented a strategy for building self-thermoregulating nanomaterials that can, in principle, be tailored to maintain a set pH, pressure, or just about any other desired parameter by meeting the environmental changes with a compensatory chemical feedback response.
Called SMARTS (Self-regulated Mechano-chemical Adaptively Reconfigurable Tunable System), this newly developed materials platform offers a customizable way to autonomously turn chemical reactions on and off and reproduce the type of dynamic self-powered feedback loops found in biological systems. The advance represents a step toward more intelligent and efficient medical implants and even dynamic buildings that could respond to the weather for increased energy efficiency. The researchers also expect that their methodology could have considerable potential for translation into areas such as robotics, computing, and healthcare.
Structurally, SMARTS resembles a microscopic toothbrush, with bristles that can stand up or lie down, making and breaking contact with a layer containing chemical “nutrients.”
“Think about how goose bumps form on your skin,” explains lead author Joanna Aizenberg, Amy Smith Berylson Professor of Materials Science at the Harvard School of Engineering and Applied Sciences (SEAS) and a Core Faculty Member at the Wyss Institute for Biologically Inspired Engineering at Harvard. “When it is cold out, tiny muscles at the base of each hair on your arm cause the hairs to stand up in an insulating layer. As your skin warms up, the muscles contract and the hairs lie back down to keep you from overheating. SMARTS works in a similar way.”
Natural materials like skin are incredibly dynamic and can maintain control in a wide range of environments through self-regulation. By contrast, synthetic materials cannot easily replicate homeostasis. Even the “smartest” materials—like eyeglasses that darken in sunlight, or a piezoelectric sensor that converts the vibrations of an acoustic guitar into a digital audio signal—typically only react to one specific environmental stimulus and do not self-regulate.
“By building dynamic feedback loops into SMARTS from the bottom up, we were able to integrate the desired regulatory features into the material itself,” says co-lead author Ximin He, a postdoctoral fellow in the Aizenberg lab at SEAS and at the Wyss Institute. “Whether it is the pH level, temperature, wetness, pressure, or something else, SMARTS can be designed to directly sense and modulate the desired stimulus using no external power or complex machinery, giving us a conceptually new robust platform that is customizable, reversible, and remarkably precise.”
To demonstrate SMARTS, He, Aizenberg, and the team chose temperature as the stimulus and embedded an array of tiny nanofibers, akin to little hairs, in a layer of hydrogel. The hydrogel, similar to a muscle, can either swell or contract in response to changes in the temperature.
When the temperature drops, the gel swells, and the hairs stand upright and make contact with the nutrient layer; when it warms up, the gel contracts, and the hairs lie down. The key aspect is that molecular catalysts placed on the tips of the nanofibers can trigger heat-generating chemical reactions in the nutrient layer.
“The bilayer system effectively creates a self-regulated on-and-off switch controlled by the motion of the hairs, turning the reaction on and generating heat when it is cold. Once the temperature has achieved a pre-determined level, the hydrogel contracts, causing the hairs to lie down, interrupting further generation of heat. When it cools again below the set-point the cycle restarts autonomously. It’s homeostasis, right down at the materials level,” says Aizenberg.
The researchers anticipate that with further refinement the technique could be integrated into materials for medical implants to help stabilize bodily functions, perhaps sensing and adjusting the level of glucose or carbon dioxide in the blood. Furthermore, the oscillating mechanical motion of the hairs could be put to work or used for propulsion, like cilia in a living organism.
Beside its technological applications, SMARTS is also an ideal “laboratory” to study the fundamental properties of biological and chemical systems, such as how living systems are able to so efficiently convert between chemical and mechanical processes.
“We found a new way to think about materials and created a fascinating system to look at some fundamental, deep questions about how living things maintain a stable state,” says Aizenberg.
Aizenberg and He collaborated with Michael Aizenberg, Wyss Institute for Biologically Inspired Engineering at Harvard; Olga Kuksenok and Anna Balazs, University of Pittsburgh; and Lauren D. Zarzar and Ankita Shastri, Department of Chemistry and Chemical Biology at Harvard University.