- Viscoelastic hydrogel matches the physical properties of human organs, reducing damage and enabling better integration with tissues than current brain implants.
- Embedded electrically conductive material can record and stimulate electrical activity, and can be twisted, stretched, and folded without breaking.
- Material supported long-term growth and differentiation of brain cells (50+ days).
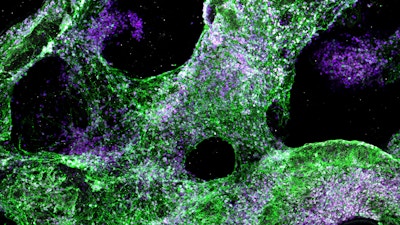
Soft hydrogel electrodes for better, safer implants
Novel conductive material safely records and stimulates electrical impulses in the brain, heart, and muscle to enable better body-machine interfaces for patient monitoring and rehabilitation
Interested in this technology?
The Wyss Institute is seeking licensing partners to help commercialize this innovation.
Immuno-Materials
Interested in this technology?
The Wyss Institute is seeking licensing partners to help commercialize this innovation.
The Problem
Brain implants are relative newcomers on the medical device scene, but are now being routinely used to record, stimulate, or block neural impulses in patients with a variety of conditions, including Parkinson’s disease and epilepsy. In order to effectively monitor or interact with brain cells, all brain implants need to contain an electrically conductive material, and the material of choice thus far has been metals. But metals are generally hard and inflexible while the brain is soft and malleable – a mismatch in physical properties that can limit implants’ ability to interface with neurons and could even cause brain damage. This is a major stumbling block in the path of the development of brain-machine interfaces and more sophisticated medical devices for monitoring, assisting, and rehabilitating the brain and other organs and tissues.
Our Solution

Researchers at the Wyss Institute have created a novel, soft electrode that marries the electrical conductivity of metal with the flexibility of a hydrogel. The alginate-based hydrogel scaffold of the electrode is embedded with a crisscrossing network of carbon nanotubes and graphene flakes, which form a continuous, conductive pathway that electrons can traverse. When placed onto tissue mimicking the human brain, the hydrogel electrode “flows” to fit its many nooks and crannies, and retains its shape after removal. It can monitor electrical activity in the brain, heart, muscle, and potentially other organs. When formed into a porous scaffold, the hydrogel material supports the growth and differentiation of human neural progenitor cells (NPCs) into multiple different brain cell types with no evidence of stress or damage to the cells.
Product Journey
Members of the Mooney lab at the Wyss Institute have spent years developing alginate hydrogels that can be used for a number of functions including surgical adhesives and single-cell encapsulation. One of these hydrogels’ properties is that they are viscoelastic, meaning that they will spring back to their original shape if pressure is applied and then released, but will deform permanently into a new shape if pressure is continuously applied. Most of the human body’s tissues are also viscoelastic, and Core Faculty member Dave Mooney and former graduate student Christina Tringides reasoned that their hydrogels could be used to make devices that can more seamlessly interact with organs. Given her background in neural engineering, Tringides decided to create hydrogel electrodes for safer and more effective monitoring of brain functions. Current standard electrode arrays are made primarily of metals and can be up to a million times stiffer than the brain, putting patients at risk of injury and reducing the accuracy of data gathered.

The hydrogel electrode uses a network of graphene flakes and carbon nanotubes as its electrically conductive component, is highly flexible and durable, and is fully biocompatible. The material can be bent more than 180 degrees, tied into knots, and stretched up to 10 times its length without breaking or tearing. The team tested their electrode on organs of various sizes, all of which experienced no damage or slipping of the device. When attached to a mouse’s heart and a rat’s brain during surgeries, the electrode successfully recorded the electrical activity of the organs and caused no injury to the animals during use.
To further expand the electrode’s capabilities, the team then created a porous scaffold made from the same conductive hydrogel material, and cultured human neural progenitor cells (NPCs) within it. The NPCs formed networks of lattice-like structures and differentiated into multiple cell types including neurons and neuron-supporting cells such as astrocytes and oligodendrocytes after five weeks. When electrical pulses were applied regularly to the brain cells through the porous scaffold, they thrived for up to 51 days. The oligodendrocytes also formed a protective myelin sheath around neurons, which has been difficult to achieve in vitro.
These soft hydrogel devices could enable the creation of better brain-machine interfaces that more seamlessly integrate with brain tissue and reduce the potential for injury during monitoring and treatment. They could help restore function in human patients who are suffering from numerous neurological and physiological problems, and could also be developed into electrode arrays for large-scale surface brain recordings and dynamic cardiac mapping. In addition, they can serve as a tool for better understanding how organs develop, function, and change throughout disease.
This technology is available for licensing.
Interested in this technology?
The Wyss Institute is seeking licensing partners to help commercialize this innovation.